지구성 운동과 메스암페타민 투여가 수컷 Sprague-Dawley 쥐의 해마에서 혈뇌장벽 관련 인자 및 BDNF 발현에 미치는 영향
Effect of Endurance Exercise and Methamphetamine Administration on the Expression of Blood-Brain Barrier Related Factors and BDNF in Hippocampus of Male Sprague-Dawley Rat
Article information
Trans Abstract
PURPOSE
This study aimed to investigate the effect of short-term endurance exercise and methamphetamine administration on bloodbrain barrier (BBB)-related marker and brain-derived neurotrophic factor (BDNF) in the hippocampus of male Sprague-Dawley (SD) rats.
METHODS
Forty male SD-rats were randomly assigned to 4 groups and treated for 2 weeks. For the methamphetamine group, 1 mg/ kg (+)-S-Methamphetamine hydrochloride (METH) was intraperitoneally injected daily, and an endurance exercise was performed at 21 m/min for 60 minutes. All treatments were performed daily, and METH or saline was administered after exercise. After 2 weeks of treatment, the head was decapitated under anesthesia, and the hippocampus was separated for western blotting.
RESULTS
Two weeks of endurance exercise significantly increased the expression of occludin and claudin-5 in the hippocampus of the rats. Similarly, phosphorylation of CREB/BDNF and AKT/GSK3 signaling pathways were significantly increased. This phenomenon was also observed in the exercise and METH co-treatment groups. However, the single treatment of 1 mg/kg METH did not affect tight junction protein and BDNF expression.
CONCLUSIONS
Endurance exercise increased the expression of BBB-related proteins and neurogenesis-related molecules, which were not inhibited by METH administration.
서 론
메스암페타민(methamphetamine, methylamphetamine, desoxyephedrine, METH)은 히로뽕 또는 필로폰으로 불리는 중추신경 흥분제로 자율 신경계의 교감 반응(빈맥, 빈호흡, 고혈압, 동공 확장, 고열, 피로 감소)과 관련된 단기 증상을 유발함과 동시에 행복감, 타인과의 관계에 대한 사회적 용이함, 주의력 증가, 행동 억제, 식욕 감소, 에너지 증가, 성욕 및 자신감 증가 등을 나타낸다[1]. 그러나 METH의 오남용은 중추 신경계(CNS)를 교란시켜 신경 정신학적 변화를 발생시키고 심각한 경우 사망에 이르게 한다[2,3]. METH는 장기간의 금욕에도 불구하고 쉽게 재발하게 되는데 이는 금단 시 불안 및 우울감이 증가할 뿐만 아니라[4,5] 복용에 의한 신경 정신학적 변화가 금단 초기 또는 금단 기간 내내 지속되기 때문이다[6].
약물장애로 고통 받고 있는 사람들은 점차적으로 증가하고 있으며, 이러한 확산은 세계적인 추세이다. 그러나 중독 치료를 위한 효과적인 치료법은 아직 제시되지 않고 있다. 현재 마약 중독 치료는 약물 치료, 인지행동치료, 사회문화 치료 등이 사용되고 있다. 그러나 이러한 치료법에는 한계가 있는데 METH 중독과 같은 약물 남용 장애는 중독의 여러 단계에서 신경생물학적 변화가 지속적으로 나타남으로 단일 약리학적 개입은 중독이 진행되는 동안 충분한 치료법이 되지 않을 수 있다[7,8]. 또한 METH 중독으로부터 회복을 위해서는 상당히 긴 시간이 필요하고, 빈번하게 재발되는 특징이 있어 행동 치료법으로는 효과적으로 치료되기 어렵기 때문에 METH 중독 관리에 대한 새로운 접근 방식이 시급히 모색되어야 한다[9].
운동은 우울증, 중독, 정신 분열증과 같은 부정적인 증상을 포함한 여러 정신 질환의 치료법으로 제안되고 있다[10-12]. 운동은 비용이 적게 들고, 실행이 용이하며, 부작용이 적고 환자들의 거부감이 낮다는 점에서 더욱 매력적인 중재법이라 할 수 있다[13]. 특히 약물 중독자의 경우 운동은 약물의 시작 단계, 장기간의 중독, 금단 및 재발 전반에 걸쳐 약물 사용의 범위, 기간 및 빈도를 저하시키는 것으로 보고되었다[14]. 또한 METH의 경우에도 신체 운동은 중독자들의 신체 건강은 물론이고 심리적 건강을 증진시켜 중독자들의 약물 재사용을 줄이는데 유용한 것으로 보고되었다[7,15,16]. 그러면 운동으로 유발되는 어떠한 신체적 변화가 이러한 긍정적인 효과를 나타내는 것일까? 아직 이에 대한 명확한 답은 없다. 선행연구들에 의하면 신체 운동을 통한 METH 사용자의 신경 생물학적 변화는 CNS neurochemicals의 조절[14,17]과 항산화제의 방어 메커니즘 개선[18-21], 신경 발생[22], 신경교형성[23] 및 뇌혈관장벽의 보호[24,25]을 포함 다양한 변화의 상호 작용 때문일 것으로 생각되고 있다.
최근 들어 뇌혈관장벽(Blood-brain barrier, BBB)에 관한 관심이 증대되고 있는데, BBB는 뇌의 항상성을 조절하기 위해 독성/병원성 화합물 및 정상적인 혈액 구성의 변화로부터 뇌를 보호함으로 중추신경을 보호하는 역할을 하는 조직이다[26]. 세포 수준에서 BBB는 기저판, 혈관주위세포, 성상세포 말단으로 둘러싸인 내피 세포(endothelial cells, EC)로 구성되며, 주변은 신경혈관 단위를 형성하는 뉴런과 미세아교 세포로 치밀하게 에워싸여 있다[27]. 이때 선택적 물질 수송은 밀접 접합(tight junction, TJ) 또는 접착 접합(adhesion junction, AJ)을 통한 인접한 EC들 사이의 세포 간 접합으로 조절된다[28]. TJ는 claudins, occludin 및 junctional adhesive molecule (JAM)과 같은 막관통 단백질과 zonula occludens (ZO), cingulin, AF-6 및 7H6을 포함한 세포질 단백질에 의해 형성되고, AJ는 막횡단 단백질인 cadherins 과 접합부의 세포질 구성요소인 catenin으로 구성된다[29]. 선행 연구에 의하면 METH와 관련된 신경 독성이 뇌 환경의 고유한 기능에 매우 중요한 BBB 기능을 손상시킬 수 있는 것으로 제안되었다. METH는 BBB 기능 장애와 고열을 통해 뇌 항상성에 부정적인 영향을 미치는데[30], claudin-5와 occluding, ZO-1을 포함한 주요 TJ 단백질의 발현 감소와 단편화, 재분배를 유도함으로 내피 TJ 조립을 붕괴하여 내피 장벽의 견고성을 감소시키게 됨으로 BBB의 투과성을 증가시키게 된다[28,31-36]. 이때 지구성 운동은 BBB의 기능을 정상화 시키는 것으로 보고되고 있는데, 저/중강도의 운동은 TJ 단백질의 발현을 증가시킴으로 BBB의 투과성을 정상화시키며[37], 다발성 경화증에서 신체 운동은 활성 산소 종의 생성과 산화 스트레스 유도를 억제함으로써 마우스의 척수에서 claudin-4 및 occludin 수준을 유지하는 것으로 보고되었다[38]. 또한 Park 등[24]은 METH 노출 후 실시된 운동(휠 러닝)이 BBB를 안정시키고 신경 발생에서 METH로 인한 부정적인 변화로부터 뇌를 보호한 것으로 보고하였다. 그러나 선행연구들의 대부분은 METH 투여 전 장기간의 운동 트레이닝 후 과량의 METH를(>10 mg/kg) 1회 투여하여 BBB의 변화를 관찰함으로 실제 마약 중독자들의 치료환경과는 차이가 있다. 이에 본 연구는 저량의 METH (1 mg/kg)와 지구성 운동의 병행이 해마 내 BBB 기능과 신경성장인자에 미치는 영향을 규명함으로 운동이 약물 중독 치료 및 재발 방지를 위한 ‘Exercise Pill’로서의 가능성을 제시해 보고자 하였다.
연구 방법
1. 연구 대상
본 연구는 10주령의 수컷 Sprague–Dawley 쥐(Hyochang science, Daegu, Korea) 40마리를 구입하여 1주간의 환경 적응 기간을 거친 후 4집단으로 무선 배정하여 2주간 처치하였다. 사료(carbohydrate 79.6%, fat 4%, protein 16.4%, #2016 Harlan Teklad, USA)와 물의 섭취는 자유롭게 하였다. 쥐는 한 케이지(20.7×35×17 cm)에 2마리씩 넣어 사육하였고, 사육실의 온도는 21°C로 유지하였다. 명기와 암기를 각각 12시간으로 조절하며 암기는 오전 7:00시-오후 7:00시로 조절하였다. 실험기간 동안 체중과 사료 섭취량은 3일 간격으로 동일 시간대에 측정하였다. 본 연구는 계명대학교 동물연구위원회의 승인을 받아 실시되었다 (KM2020-008).
• Control group (CON): saline injection, i.p, 7 day/week, n=10
• Methamphetamine injection group (MA): 1 mg/kg/day METH-HCl, i.p, 7 day/week, n=10
• Exercise training group (Ex): saline injection, treadmill running, 21 m/min, 60 minutes, slope 0%, 7 day/week, n=10
• Methamphetamine injection + Exercise training group (ME): n=10
2. 메스암페타민 및 운동처치
1) 메스암페타민 투여
(+)-S-Methamphetamine hydrochloride (M8750, Sigma-Aldrich, USA)를 0.9% 멸균 식염수에 녹여 최종 농도가 0.6 mg/mL가 되도록 하였다. METH 투여 집단은 1 mg/kg/day METH-HCl을, 식염수 투여집단은 동일양의 식염수를 복강에 투여하였다[39,40]. 모든 약물은 사용 전에 신선하게 준비하였고, 14일간 1일 1회 동일한 시간대에 투여하였다. ME집단은 운동직후 METH를 투여하였다[41].
2) 운동 트레이닝
지구성 운동은 실험동물용 전동 트레드밀(FT-200, Techman Soft)을 이용하여 2주간 1일 1회 실시하였다. Wang 등[42]은 METH 중독자의 약물 갈망 행동이 중간 강도의 운동에서 가장 효과적으로 감소되었다고 보고했다. 이에 Wang 등[42]과 Kemi 등[43]의 연구 방법을 부분적으로 수정하여 중강도(60% VO2max, 21 m/min, 경사 0%)로 1일 60분간 트레밀을 달리도록 구성하였다. 운동강도는 초기 12 m/min, 15분에서 시작하여 점진적으로 시간과 속도를 증가시켜 1주일 후에는 목표 운동강도로 운동을 할 수 있도록 하였다.
3. 실험 절차
총 처치기간은 14일로 모든 처치는 오전 9-12시에 시행하였다. ME 집단은 트레드밀 달리기 후 METH를 투여하였다[41]. 처치 종료일(14일)에는 METH 또는 생리식염수 주사 후 운동처치 없이 2시간 휴식 후 마취(50 mg/kg pentobarbital sodium, i.p.) 하였다. 약 20분 후 plantar flex test로 완전히 마취됨을 확인한 후 배를 열어 복대정맥으로 혈액을 모두 체취하고 머리를 분리하였다[44]. 분리된 머리는 얼음 위에서 신속하게 좌, 우 해마(hippocampus)를 분리하여 액체 질소로 즉시 동결한 후 분석 전 까지 -80°C에서 보관하였다.
4. 분석
해마는 ice-cold RIPPA buffer (250 mM sucrose, 10 mM HEPES/1 mM EDTA (pH 7.4), 1 mM Pefabloc (Roche), 1 mM EDTA, 1 mM NaF, 1 g/mL aprotinin, 1 g/mL leupeptin, 1 g/mL pepstatin, 0.1 mM bpV (phen), 2 mg/mL glycerophosphate 함유, 4°C)를 사용하여 handy vortex mixer (T24-132-500, Lklab Korea)로 30초간 균질화 하였다. 균질화된 시료는 동결/해동 과정을 3회 반복한 다음 10분간 원심분리(700 g, 4°C)하여 상층액만 사용하였다. 단백질 농도는 Lowry 등[45]의 방법에 의해 정량분석 하였다. 정량분석 후 시료는 laemmli sample buffer (100 mM dithiothreitol 함유)를 첨가하여 5분간 가열하여 용해한 후 사용하였다. 준비된 시료는 SDS-polyacrylamide gel을 이용하여 전기영동한 후 nitrocellulose membrane에 transfer 하였다. Membrane은 5% nonfat dry milk로 1시간 동안 blocking하고, TBST (TBS+0.1% Tween-20, pH 7.5)로 세척한 다음 일차항체와 함께 배양(overnight, 4°C) 하였다. Immunoblotting을 위해 사용된 일차항체는 다음과 같다: Claudin-5 (1:500, Invitrogen, # 35-2500), GSK3β (1:1,000, Santa Cruz Bio, sc-81462), phospho (Ser9)-GSK3β (1:1,000, Santa Cruz Bio, sc-373800), phospho (Ser473)-AKT (1:1,000, Cell signaling, #4060), AKT (1:1,000, Cell signaling, #2920), phospho-CREB (1:500, Invitrogen, #MA5-11192), CREB (1:500, Invitrogen, #MA1-083), BDNF (1,000, Invitrogen, #PA1-18357), β-actin (1:1,000, abcam, ab106814). 각각의 2차 항체 처리(60 minutes, 상온) 후 ECL (Amersham)을 이용해 시각화하고 densitometry (sigma-plot 8.0 system)로 정량하였다. Loading control은 β-actin으로 하였고, 목표 단백질 발현 수준은 β-actin 발현수준에 대해 표준화되어 arbitrary units (AU)로 표시하였다. 인산화에 의해 활성화되는 단백질은 총 단백질 발현량에 대한 인산화 수준의 상대적 비율로 제시하였다.
5. 자료 처리
각 측정항목에 대한 결과는 평균과 표준오차(Mean±SE)로 산출하였고, 통계적 분석은 SigmaPlot 12.0 통계패키지를 이용하였다. 집단간 차 이를 검증하기 위해 일원분산분석(one-way ANOVA)를 실시하였고, 사후검정은 Tukey법을 이용하였다. 통계적 유의수준은 α=.05로 하였다.
연구 결과
1. 2주간 지구성 운동 또는 METH 투여는 식이 섭취와 체중 증가에 영향을 미치지 않았다
2주간의 중강도 지구성 운동과 METH 처치는 수컷 SD 쥐의 식이섭취량과 체중에 유의한 영향을 미치지 않았다(Fig. 1A, B).
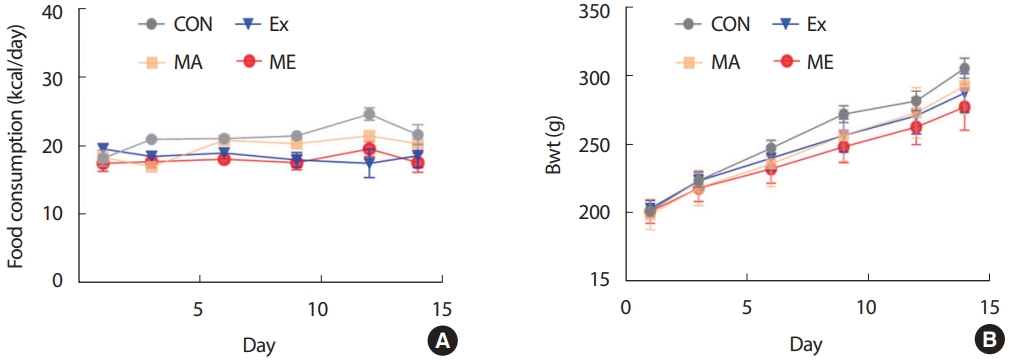
Endurance exercise or METH injection did not affect dietary intake and weight gain during the study period. (A) Food consumption, (B) Body weight, Value are means±S. CON, saline control (n=10); MA, METH injection group (n=10); Ex, endurance exercise training group (n=10); ME, METH plus exercise training group (n=10).
2. 지구성 운동은 해마내 TJ 단백질 발현을 유의하게 증가시켰다
수컷 SD 쥐의 해마내 TJ 단백질인 claudin-5와 occludin의 단백질 수준은 CON과 MA 집단에 비해 Ex, ME 집단에서 유의하게 증가하였다 (p<.05). CON 집단과 MA 집단, Ex와 ME 집단 간에는 claudin-5와 occludin 단백질 수준의 유의한 차이가 나타나지 않았다(Fig. 2A, B).

Endurance exercise training significantly increased TJ protein level in hippocampus of rats. (A) Claudin-5/β-Actin ratio, (B) Occludin/β-Actin ratio, (C) p/t Akt ratio, (D) p (ser9)/t GSK3β ratio, Value are means±SE. CON, saline control (n=10); MA, METH injection group (n=10); Ex, endurance exercise training group (n=10); ME, METH plus exercise training group (n=10). *p<.05, **p<.01.
조직 내 TJ 단백질의 양적 증가는 생성의 증가와 분해의 감소 측면을 모두 의미한다. 선행연구에 의하면 GSK3β의 저해는 TJ 단백질의 반 감기를 증가시키는 것으로 보고되었는데[46], 본 연구의 결과에서도 Ex, ME 집단의 GSK3β와 상위 신호인자인 Akt의 인산화가 CON, MA 집단에 비해 유의하게 증가하였다(p<.05, Fig. 2C, D).
3. 지구성 운동은 해마 내 BDNF 발현을 유의하게 증가시켰다
본 연구의 결과 2주간의 지구성 운동은 쥐의 BDNF 발현을 유의하게 증가시켰고, 이러한 현상은 ME집단에서도 동일하게 나타났다(p<.05, Fig. 3B). BDNF의 발현을 유도한 신호전달체계를 알아보기 위해 상위 신호인자들을 분석한 결과 Ex, ME 집단의 해마 내 CREB 인산화가 CON과 MA 집단에 비해 유의하게 증가하였다(p<.05, Fig. 3A). CON과 MA 집단 간, Ex와 ME 집단 간의 BDNF 발현의 유의한 차이는 나타나지 않았다.

Endurance exercise training significantly increased BDNF expression in hippocampus of rats. (A) p/t CREB ratio, (B) BDNF/β-Actin ratio, Value are means±SE. CON, saline control (n=10); MA, METH injection group (n=10); Ex, endurance exercise training group (n=10); ME, METH plus exercise training group (n=10). *p<.05, **p<.01.
논 의
필로폰 중독과 같은 약물 남용 장애는 중독의 여러 단계에서 신경 생물학적 변화가 지속적으로 나타나기 때문에 단일 약리학적 개입은 중독이 진행되는 동안 충분하지 않다[7,8]. 운동 요법은 비용이 적게 들고, 실행이 용이하다. 운동은 악물의 시작 단계, 장기간의 중독, 금단 및 재발 전반에 걸쳐 약물 사용의 범위, 기간 및 빈도와 부적 상관관계를 갖는 것으로 보고되어 잠재적인 비약물적 치료법으로 제안되고 있다[14]. 선행연구에 따르면 METH 중독자들의 중강도 운동 트레이닝은 우울증을 현저하게 낮추고, 약물중독자를 위한 단체 운동은 이들의 신체 건강과 심리적 측면을 크게 개선시킨 것으로 나타났다[15]. 그러나 아직 METH로 유도되는 신경생물학적 변화에 신체 운동이 미치는 잠재적인 영향에 대한 연구는 매우 부족하다. 이에 본 연구는 규칙적인 중강도 지구성 운동과 저용량의 METH가 해마 내 BBB 관련 인자들과 BDNF 발현에 미치는 영향을 규명함으로 METH 오남용의 치료제로 운동의 효과를 증명해 보고자 하였다. 본 연구의 목적을 위해 SD rat을 대상으로 2주간 중강도의 지구성 운동과 1 mg/kg의 METH 투여를 병행한 후 뇌의 해마에서 TJ 단백질과 BDNF의 발현과 이들 인자들의 상위 신호분자들의 변화를 분석하였다. 연구 결과 2주간의 1 mg/kg METH 처치는 쥐의 해마 내 occludin과 claudin-5의 발현에 영향을 미치지 않았다. 그러나 지구성 운동은 TJ 단백질인 occludin과 claudin-5의 발현을 유의하게 증가시켰고, METH 병행 처치집단에서도 유사한 결과가 나타났다. 본 연구의 결과와 유사하게 Lafuente 등[47]은 규칙적인 수영이 수컷 SD 쥐의 METH 유발 뇌손상과 BBB 누출을 감소시킨 것으로 보고하였다. 또한 중등도의 유산소 운동은 뇌미세혈관에서 METH 유발 산화 스트레스를 약화시키고 모세혈관의 항산화 능력을 높여 METH에 의한 BBB 파괴로부터 보호하는 것으로 나타났다[42]. 비록 본 연구에서 METH처치에 따른 TJ 단백질 발현의 감소는 관찰되지 않았으나, 지구성 운동은 단독 또는 METH 병행에도 해마내 TJ 단백질의 발현을 유의하게 증가시킴으로 METH 유발 BBB의 붕괴에도 지구성 운동의 병행은 BBB 기능 유지에 긍정적인 영향을 미칠 것으로 생각한다. 한편 지구성 또는 저항성 운동은 GSK3β의 인산화를 촉진하여 활성을 억제함으로 BBB 강화와 복구, 보호하는 역할을 하는 것으로 알려져 있다[48]. GSK-3은 안정시에 활성화 되고, 인산화(ser9-GSK3β 및 ser21-GSK3α)함으로 저해되는데, 다양한 kinase (tyrosine kinase, G-protein couple receptors, Akt 등)에 의해 GSK-3은 인산화 된다[49]. Akt는 Thr308과 Ser473 [50]의 인산화에 의해 활성화되어 GSK3β를 직접적으로 인산화함으로 GSK3β를 저해하게 된다[51]. Ramirez 등[52]은 자발적인 지구성 운동이 occludin과 claudin-5의 반감기를 증가시킴으로 내피 세포에서 TJ 안정성을 촉진하는 것으로 보고하였고, GSK3β recruitment를 감소시킴으로 TJ 안정성을 유도하여 BBB를 보호하였다[46]. 또한 GSK-3β의 억제는 뇌내피세포에 대한 항 염증 효과가 있어 BBB 보호에 긍정적인 영향을 미치는 것으로 보고되었다[53]. 본 연구의 결과 지구성 운동은 해마 내 AKT/GSK3β의 인산화를 증가시킴으로 GSK3β의 활성을 억제하였고, METH 병행처치 집단에서도 이러한 효과는 동일하게 나타났다. 따라서 본 연구결과 나타난 TJ 단백질의 발현 증가는 지구성 운동의 직접적인 TJ 단백질의 발현 증가 또는 GSK3β 저해를 통한 TJ 단백질의 반감기 증가 때문으로 생각된다.
본 연구결과 2주간의 지구성 운동은 해마 내 CREB/BDNF의 발현을 유의하게 증가시켰다. BDNF는 신경 발달, 생존 및 이동, 신경 발생, 세포 분화, 축삭 및 수상돌기의 성장, 시냅스 형성 및 시냅스 가소성에 필수적인 인자로[54-56], 학습과 기억에 중요한 역할을 하는 해마에서 고도로 발현되는데, 해마는 다양한 자극에 쉽게 손상되는 조직으로 여러 신경퇴행성 장애 시 가장 초기에 영향을 받는 곳이기도 하다[57]. 다수의 선행연구에 의하면 규칙적인 운동은 해마에서 BDNF의 발현을 유도하여 해마 의존적 기억력을 향상시키는 것으로 보고되었다[58-61]. 동물 모델에서 자발적인 휠 운동은 해마 내 BDNF 발현을 증가시키고, 동시에 기억력과 인지력이 증가한 것으로 나타나 운동을 통한 인지력과 기억력증가는 해마 내 BDNF의 발현과 밀접한 상관성이 있는 것으로 여겨지고 있다[62,63]. 그러나 본 연구에서 인지력과 기억력을 측정하지 못하였기 때문에 인과관계를 논할 수는 없으나 지구성 운동을 통한 해마 내 CREB/BDNF 발현의 증가는 METH유발 뇌신경 퇴화의 회복 또는 예방에 긍정적인 영향을 미칠 수 있을 것으로 생각된다.
본 연구자는 SD 쥐를 대상으로 2주간 지구성 운동 또는 METH 투여후 행동양상 및 선조체내 보상관련 신호전달 인자를 분석한 결과 지구성 운동은 METH 유발 흥분성을 유의하게 감소됨을 보고한바 있다[41]. 이러한 선행연구를 바탕으로 중정도의 지구성 운동과 METH 중독자들이 일반적으로 복용하는 양(~1 mg/kg)을 적용하여 BBB 기능에 미치는 영향을 규명하고자 하였다. 그러나 본 연구에서 METH에 의한 BBB 붕괴와 신경성장인자의 저해는 나타나지 않았다. Martins 등[64]은 30 mg/kg METH의 투여 후 마우스 해마에서 TJ 단백질(claudin-5, occluding, ZO-1)이 감소하여 BBB의 투과성이 증가한 것으로 보고하였다. 또한 3주간 2.5-10 mg/kg METH를 점증적으로 증량하여 투여한 결과 occludin 및 ZO-1의 발현이 감소하여 BBB가 손상된 것으로 나타났다[65]. 이를 통해 본 연구에서 사용된 METH의 양이 선행연구에 비해 매우 적은 양이었기 때문에 BBB 손상이 나타나지 않은 것으로 생각된다. 때문에 본 연구에서 지구성 운동이 METH 유발 BBB 기능 저하에 미치는 영향을 결론 내릴 수는 없으나, 선행연구의 결과에 비추어 볼 때 지구성 운동과 METH의 병행처치는 METH 유도 BBB 기능 저하를 예방할 수 있을 것으로 생각된다. 이러한 부분은 추후 연구를 통해 반드시 규명될 필요가 있다.
결 론
2주간의 규칙적인 중강도 지구성 운동은 해마내 TJ 단백질과 BDNF의 발현을 증가시켰으나 1 mg/kg METH 처치는 영향을 미치지 않았다. 그러나 METH와 지구성 운동 병행은 운동 단독처치와 유사하게 TJ 단백질과 BDNF 발현을 증가시켰다. 이를 통해 중강도의 지구성 운동은 해마의 장벽 기능과 신경성장을 증가시킴으로 뇌 보호에 긍정적인 영향을 미칠 것으로 생각된다. 그러나 본 연구에서 METH 처치에 의한 인자들의 부정적 변화가 관찰되지 않았기 때문에 지구성 운동이 METH 유발 뇌기능 저하에 영향을 미친다고 단정 내릴 수 없다. 따라서 추후 METH 투여량에 따른 운동의 효과를 규명할 필요가 있다.
Notes
이 논문 작성에 있어서 어떠한 조직으로부터 재정을 포함한 일체의 지원을 받지 않았으며, 논문에 영향을 미칠 수 있는 어떠한 관계도 없음을 밝힌다
AUTHOR CONTRIBUTION
Conceptualization: SR Jung; Data curation: SR Jung; Formal analysis: SR Jung; Funding acquisition: SR Jung; Methodology: SR Jung; Project administration: SR Jung; Visualization: SR Jung; Writing-original draft: SR Jung; Writing-review & editing: SR Jung.